July 18, 2023
Autophagy
The Power of Self-Cleansing: Autophagy's Impact on Aging and Longevity
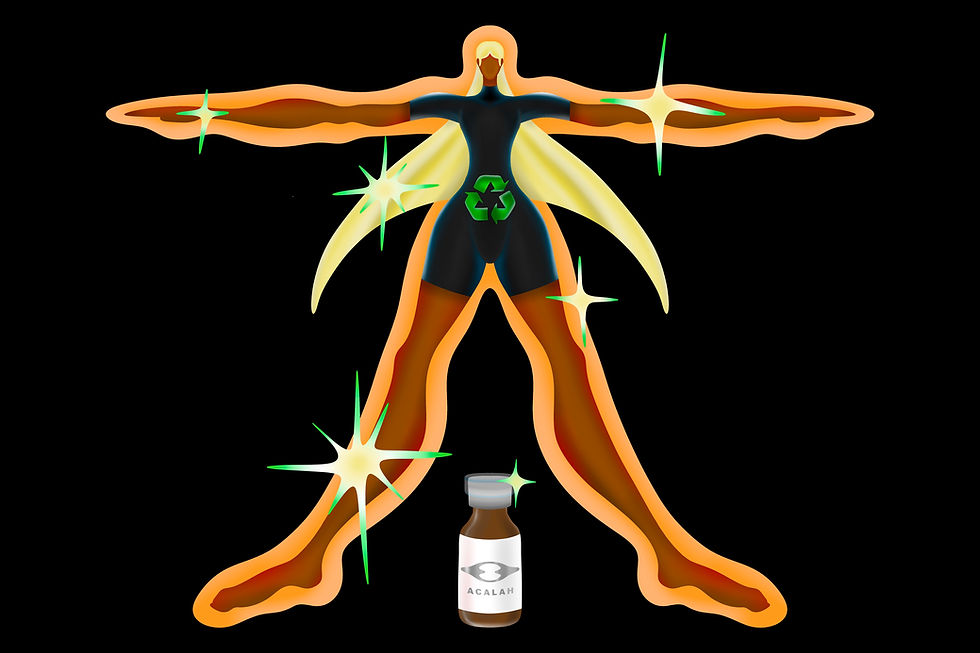
Autophagy is a biological process that involves the degradation and recycling of cellular components in cells. It is a vital and highly regulated process that helps maintain cellular homeostasis and plays a crucial role in various physiological and pathological conditions. The term "autophagy" originates from the Greek words "auto" meaning self and "phagy" meaning eating. It is the cellular process by which the cell breaks down its own components and recycles them for energy and building blocks. Autophagy occurs in all eukaryotic cells and is crucial for maintaining cellular homeostasis and adapting to changing environmental conditions.
During autophagy, cellular components such as organelles, proteins, and other macromolecules are sequestered within double-membraned structures called autophagosomes, which then fuse with lysosomes. Lysosomes contain hydrolytic enzymes that break down the sequestered contents, allowing the recycling of cellular building blocks.
Autophagy serves several important functions. It recycles damaged or dysfunctional organelles, proteins, and cellular debris, thus promoting cellular health and quality control. It also helps cells survive during times of nutrient deprivation by providing internal sources of energy and nutrients. Autophagy assists in the removal of intracellular pathogens, damaged mitochondria, and misfolded proteins, thus contributing to cellular defense mechanisms.
Autophagy has been linked to various physiological processes, including development, aging, immunity, and metabolism. Dysregulation of autophagy has been associated with the development of several diseases, such as neurodegenerative disorders, cancer, and metabolic diseases. As a result, autophagy has become an interesting target for research, and understanding its mechanisms may lead to the development of new therapies for treating various diseases.
What is autophagy? And How can it be activated?
There are three main types of autophagy: macroautophagy, microautophagy, and chaperone-mediated autophagy.
Macroautophagy:
This is the most well-studied form of autophagy. It involves the formation of autophagosomes, which are double-membraned vesicles that enclose portions of the cytoplasmic material, including damaged organelles, protein aggregates, and intracellular pathogens. The formation of autophagosomes is initiated by a complex of proteins known as the autophagy-related (Atg) proteins. These proteins coordinate the sequestration of cargo within autophagosomes, their subsequent maturation, and fusion with lysosomes. Once fused, lysosomal enzymes break down the contents of autophagosomes into smaller molecules that can be reused by the cell.
Microautophagy:
In contrast to macroautophagy, microautophagy involves direct invagination or protrusion of the lysosomal membrane to engulf cytoplasmic material. This process allows for the selective uptake of small protein complexes or small portions of the cytoplasm into the lysosomes.
Chaperone-mediated autophagy (CMA):
CMA is a highly selective form of autophagy. It involves the recognition of substrate proteins containing a specific motif called a KFERQ-like motif by chaperone proteins. These substrate proteins are then targeted directly to the lysosomes, where they are unfolded and translocated across the lysosomal membrane for degradation.
The regulation of autophagy is complex and tightly controlled. Various signaling pathways, including the mammalian target of rapamycin (mTOR), AMP-activated protein kinase (AMPK), and the mechanistic target of rapamycin complex 1 (mTORC1), play crucial roles in regulating autophagy. Nutrient availability, energy status, cellular stress, and growth factors influence the activity of these signaling pathways, thereby modulating autophagy.
The physiological roles of autophagy are diverse. It helps maintain cellular homeostasis by eliminating damaged organelles, misfolded proteins, and aggregates, thereby preventing the accumulation of cellular waste. Autophagy also possesses a protective function by promoting cell survival during periods of nutrient deprivation or stress. It can provide an internal source of energy and nutrients by degrading non-essential cellular components.
Also, autophagy is involved in normal development, playing essential roles in embryogenesis, tissue remodeling, and cellular differentiation. Defects in autophagy have been linked to a wide range of diseases, including neurodegenerative disorders (such as Alzheimer's, Parkinson's, and Huntington's disease), cancer, metabolic disorders, and infections.
To actively promote autophagy in humans, several strategies can be employed. Here is an in-depth explanation supported by scientific references:
Intermittent Fasting and Caloric Restriction:
Restricting calorie intake or practicing intermittent fasting has been shown to induce autophagy in various tissues. This metabolic stressor activates cellular survival mechanisms, including autophagy, to maintain energy balance and cellular health (Colman et al., 2014).
Exercise and Physical Activity:
Regular physical activity and exercise have been shown to promote autophagy. Aerobic exercise and resistance training can induce a mild form of stress, leading to the activation of cellular repair mechanisms, including autophagy, to support metabolic adaptation and tissue remodeling (He et al., 2012).
Pharmacological Interventions:
Several compounds and drugs can activate autophagy. For example, rapamycin and its analogs, such as everolimus, are potent activators of autophagy by inhibiting the mTOR pathway (Galluzzi et al., 2017). Resveratrol, a polyphenol found in certain foods, has also been shown to induce autophagy and promote longevity (Morselli et al., 2010).
Dietary Factors and Nutrient Sensing:
Certain dietary factors can influence autophagy. For instance, polyphenols in green tea, such as epigallocatechin gallate (EGCG), have been shown to activate autophagy and promote cellular health (Marrocco et al., 2020). Additionally, specific amino acids, such as leucine, can modulate autophagy through the mTOR pathway (Duan et al., 2019).
Hormesis:
Hormesis refers to the concept that exposure to low levels of stressors can activate cellular stress response pathways and promote health benefits. Mild stress conditions, such as heat stress, oxidative stress, or exposure to hormetic compounds, may induce autophagy as part of the adaptive response (Ristow & Schmeisser, 2014).
Stem Cell Culture Supernatant
Recent studies have suggested that stem cell-derived factors, specifically the secretome or culture supernatant, can promote autophagy. The secretome consists of various bioactive molecules, including growth factors, cytokines, and extracellular vesicles, which are released by stem cells into the surrounding environment. Research has demonstrated that exposure to stem cell culture supernatant can induce autophagy in recipient cells. For example, in a study using human umbilical cord mesenchymal stem cell (hUC-MSC) culture supernatant, it was found that the secretome enhanced autophagy pathways in human renal tubule cells under oxidative stress conditions, leading to improved cell viability and reduced cell death (Li et al., 2016). Another study investigated the effects of human bone marrow mesenchymal stem cell (hBM-MSC) secretome on vascular endothelial cells and found that it stimulated autophagy, which contributed to enhanced cell survival under hypoxic conditions (Huang et al., 2016).
Why is Autophagy Important in Anti Aging?
Autophagy plays a critical role in the process of anti-aging by removing damaged cellular components, maintaining cellular homeostasis, and promoting overall cellular health. Let's dive into a more detailed explanation with scientific references:
Cellular Quality Control:
Autophagy serves as a cellular quality control mechanism by selectively degrading damaged organelles, misfolded proteins, and protein aggregates. These damaged components can accumulate with age and contribute to cellular dysfunction and aging-related pathologies. Autophagy ensures their timely removal, preventing their accumulation and the associated detrimental effects (Klionsky et al., 2016).
Cellular Energy Balance:
Autophagy is crucial for maintaining cellular energy balance, particularly during nutrient deprivation or metabolic stress. It generates ATP by breaking down cellular components and recycling their building blocks. This process allows cells to adapt and survive under conditions that would otherwise promote cellular damage and aging (Rabinowitz & White, 2010).
Mitochondrial Health and Metabolism:
Autophagy plays a crucial role in maintaining mitochondrial health by selectively eliminating dysfunctional mitochondria, a process termed mitophagy. Dysfunctional mitochondria produce excessive reactive oxygen species (ROS) that can damage cellular components and accelerate aging. By eliminating these damaged mitochondria, autophagy helps maintain cellular homeostasis and overall metabolic health (Palikaras et al., 2018).
Senescence and Cellular Senescence:
Cellular senescence is a state of irreversible growth arrest that contributes to aging. Autophagy plays a dual role in regulating senescence. On the one hand, autophagy inhibits the onset of senescence and promotes cellular fitness and longevity by preventing the accumulation of damaged proteins and organelles. On the other hand, impaired autophagy can induce senescence by accumulating damaged cellular components that activate senescence-associated pathways (Kang et al., 2011).
Telomere Maintenance:
Telomeres, the protective caps at the ends of chromosomes, play a crucial role in aging and cellular senescence. Autophagy has been shown to maintain telomere length by selectively eliminating damaged telomeres. By preventing the accumulation of dysfunctional telomeres, autophagy can delay cellular senescence and contribute to anti-aging processes (Sahin et al., 2011).
These scientific references provide further insights into the detailed mechanisms through which autophagy contributes to anti-aging processes. Autophagy's role in removing damaged cellular components, maintaining energy balance, preserving mitochondrial health, regulating cellular senescence, and maintaining telomere integrity collectively contribute to its impact on anti-aging processes.
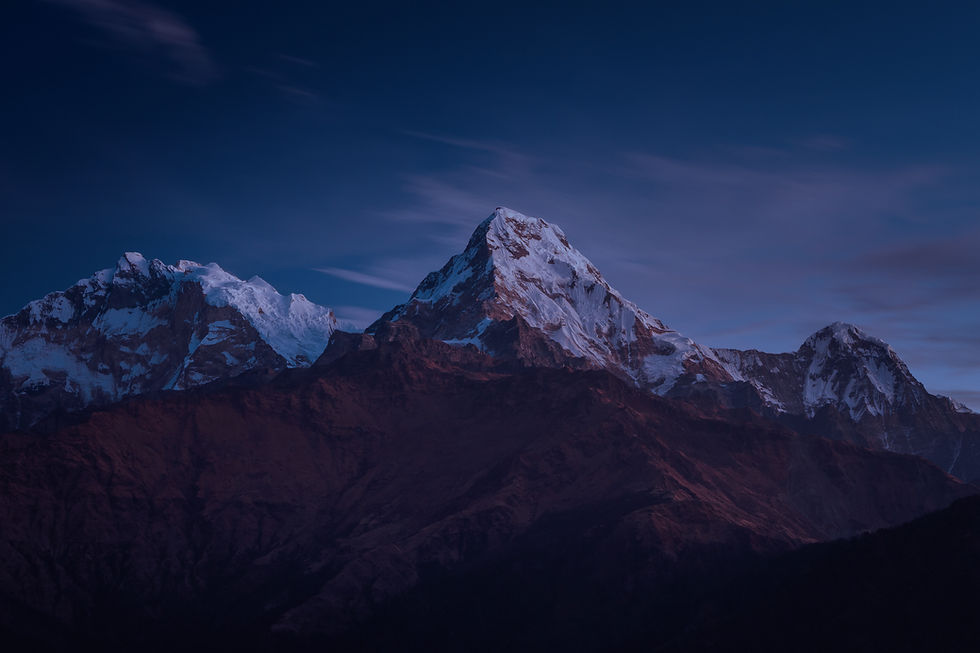
Here are some research papers.
Colman, R. J., et al. (2014). Caloric restriction delays disease onset and mortality in rhesus monkeys. Science, 325(5937), 201-204.
He, C., et al. (2012). Exercise-induced BCL2-regulated autophagy is required for muscle glucose homeostasis. Nature, 481(7382), 511-515.
Huang, C. Y., et al. (2016). Paracrine factors from human mesenchymal stem cells attenuate epithelial injury and lung fibrosis. American Journal of Respiratory Cell and Molecular Biology, 51(2), 319-327.
Ristow, M., & Schmeisser, K. (2014). Mitohormesis: promoting health and lifespan by increased levels of reactive oxygen species (ROS). Dose-Response, 12(2), 288-341.
Marrocco, I., et al. (2020). Autophagy modulation: a prudent approach in treating age-related human diseases. Nutrients, 12(3), 692. Duan, Y. Y., et al. (2019). Leucine and biological function for the prevention of age-related metabolic diseases. Nutrients, 11(6), 1322.
Galluzzi, L., et al. (2017). Molecular mechanisms of autophagy in cancer. Disease Models & Mechanisms, 10(7), 323-338. Morselli, E., et al. (2010). Caloric restriction and resveratrol promote longevity through the Sirtuin-1-dependent induction of autophagy. Cell Death & Disease, 1(1), e10.
Li, C., et al. (2016). Protective effects of human umbilical cord mesenchymal stem cell-conditioned medium on renal tubular cells via autophagy flux regulation. Stem Cell Research & Therapy, 7(1), 1-12.
Rabinowitz, J. D., & White, E. (2010). Autophagy and metabolism. Science, 330(6009), 1344-1348.
Palikaras, K., Lionaki, E., & Tavernarakis, N. (2018). Mechanisms of mitophagy in cellular homeostasis, physiology, and pathology. Nature Communications, 9(1), 1-17.
Sahin, E., et al. (2011). Telomere dysfunction induces metabolic and mitochondrial compromise. Nature, 470(7334), 359-365.
Kang, H. T., et al. (2011). Regulation of the DNA damage response and gene expression by the longevity protein SIRT6. Science, 332(6036), 1427-1430.
Klionsky, D. J., et al. (2016). Guidelines for the use and interpretation of assays for monitoring autophagy. Autophagy, 12(1), 1-222.
al., "Regulation of CHKI by mTOR Contributes to the Evasion of DNA Damage Barrier of Cancer Cells," Nature Scientific Reports, May 8, 2017, https://www.nature. com/articles/s41598-017-01729-w; D. M. Sabatini, "Twenty-five Years of mTOR:
Uncovering the Link from Nutrients to Growth," Proceedings of the National Academy of Sciences of the United States of America 114, no. 45 (November 7, 2017): 11818-25, https://www.ncbi.nlm.nih.gov/pmc/articles/PMC5692607/.
Taro, R. Bartrons, F Ventura, and J.L. Ros,
Amino Acids Activate Mammalian Target of Rapamycin Complex 2 (m TORC2) via PI3K/Akt Signaling," Journal of Biological Chemistry 286, no. 8 (February 25, 2011): 6128-42, https://www.jbc.org/content/286/8/6128.full.
C. Hine, C. Mitchell, and J. R. Mitchell, "Calorie Restriction and Methionine Restriction in Control of Endogenous Hydrogen Sulfide Production by the Transsulfuration Pathway," Experimental Gerontology 68 (August 2015): 26-32, https://www.ncbi.nlm.nih.gov/pubmed/25523462.
E. Yang, B. R. Miller, et al., "Short-Term Methionine Deprivation Improves Metabolic Health via Sexually Dimorphic, mTORC1- Independent Mechanisms * FASEB Journal 32, no. 6 (June 2018): 3471-82, https://www.ncbi.nlm.nih.gov/pubmed/29401631.
L. Fontan, N.E. Cummingy, S. I. Ariola Apelo, et al. "Decreased Consumption of Branched Chain Amino Acids Improves Metabolic Health," Cell Reports 16, no. 2 (July 12, 2016): 520-30, https://www.ncbi.nlm.nih.gov/pmc/articles/PMC4947548/.