July 3, 2023
Telomere
The Role of Telomeres in Anti-Aging Strategies: From Chromosomal Caps to Longevity
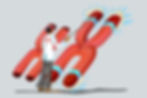
Telomeres, the protective caps located at the ends of chromosomes, are essential for maintaining cellular health and integrity. Comprised of repetitive DNA sequences and associated proteins, telomeres serve as guardians of the genome, preventing chromosomal degradation, DNA damage, and recombination errors.
The Essence of Telomeres
Telomeric DNA consists of repetitive sequences, often TTAGGG in humans, which are repeated hundreds to thousands of times. This repetitive nature enables telomeres to form unique structures that are integral to their function. Telomeres protect the ends of chromosomes by preventing them from being recognized as damaged DNA, protecting important genetic material from degradation and fusion events.
Telomeres and Cellular Health
The significance of telomeres in cellular health lies in their ability to maintain chromosome stability. During each round of cell division, telomeres undergo gradual shortening due to the end-replication problem, where the enzymes responsible for DNA replication cannot fully replicate the ends of linear chromosomes. This gradual telomere attrition serves as a cellular clock, eventually triggering a DNA damage response when telomeres reach a critically short length.
Telomere shortening and dysfunction have been implicated in various cellular processes and contribute to the aging of cells and organisms. When telomeres become too short, they signal cellular senescence - a state of permanent cell cycle arrest. This hinders the uncontrolled proliferation of damaged or dysfunctional cells that could potentially lead to the formation of cancerous tumors. Telomere dysfunction and genomic instability play a significant role in aging-related diseases and conditions.
Moreover, telomeres are involved in maintaining genomic stability and preventing chromosomal abnormalities. They ensure that chromosomes are faithfully segregated during cell division, reducing the risk of aneuploidy and other chromosomal aberrations. Telomeres also interact with different cellular components, including proteins involved in DNA repair and telomere length regulation, further highlighting their importance in maintaining cellular health.
Understanding the mechanisms underlying telomere biology is crucial for unraveling the complexities of aging, disease development, and cellular health. By studying telomeres, researchers can gain insights into the intricate interplay between these protective caps, cell cycle regulation, DNA repair, and the establishment of cellular senescence.
Telomeres serve as vital guardians of chromosomal integrity, preserving the genetic material and contributing to cellular health. Their gradual shortening acts as a cellular clock, regulating cell division and preventing the propagation of damaged cells. Telomere dysfunction, observed in aging and disease, underscores their significance in cellular processes and underscores their potential as targets for therapeutic interventions aimed at combating age-related disorders. Continued research in telomere biology holds promise for unlocking novel strategies to promote healthy aging and mitigate the impact of age-related diseases.
Telomeres: Deciphering the Mechanisms of Cellular Aging
The Biology of Telomeres
Telomeres are fascinating structures located at the ends of chromosomes that play a critical role in maintaining genomic stability and preserving cellular health. This section delves deeper into the structure of chromosomes, the unique characteristics of telomeric DNA sequences, the role of telomerase in elongating telomeres, and the importance of telomere-binding proteins in maintaining stability and functionality.
Structure of Chromosomes: Illuminating Telomeres' Perspective
Chromosomes consist of DNA molecules tightly wrapped around histone proteins, forming a complex called chromatin. At the tips of linear chromosomes, there exist regions known as telomeres that cap and protect the valuable genetic material within. Without proper protection, chromosome ends are susceptible to degradation, telomere fusion, and inappropriate recombination, which can lead to genomic instability and various diseases.
Telomeric DNA Sequences
Telomeric DNA sequences are composed of repetitive nucleotide sequences located at the ends of chromosomes. In humans, the canonical telomeric repeat sequence is TTAGGG, which is repeated in tandem hundreds to thousands of times. These repeated sequences form a distinctive structure that plays a vital role in telomere function and stability.
The Role of Telomerase: Elongating the Telomeres
Telomerase is an enzyme complex that counteracts the progressive shortening of telomeres. It consists of an RNA component called telomerase RNA (TERC) and a protein component called telomerase reverse transcriptase (TERT). Telomerase extends telomeres by adding telomeric repeats to the chromosome ends using its built-in RNA template.
While most somatic cells in the human body exhibit low or no telomerase activity, certain cell types necessitate its presence. Germ cells, stem cells, and rapidly dividing cell populations, such as those found in fetal tissues, display higher telomerase activity, ensuring the preservation and elongation of telomeres. Conversely, the downregulation of telomerase activity in somatic cells leads to telomere shortening, a characteristic associated with aging and disease progression.
Telomere Binding Proteins: Maintaining Stability
Telomere binding proteins play a crucial role in maintaining the stability and functionality of telomeres. These proteins specifically recognize and bind to telomeric DNA sequences, forming a protective cap that shields telomeres from degradation and inappropriate DNA repair responses.
One prominent group of telomere binding proteins is shelterin, a multi-subunit complex essential for telomere protection and regulation. Shelterin components include Telomeric Repeat-binding Factors (TRFs), Protection of Telomeres (POT1), Telomere Repeat-binding Factor Interacting Protein 1 (TIN2), Repressor/activator Protein 1 (RAP1), and TPP1 (also known as TINT1/PTOP or PIP1). Together, these proteins facilitate the organization and stabilization of telomeres, preventing them from being recognized as damaged DNA and regulating telomere lengthening processes.
Mechanisms of Telomeres
Telomeres undergo dynamic processes that are crucial for their replication, maintenance, and function. Understanding these mechanisms is essential for comprehending the impact of telomere shortening and dysfunction on cellular senescence, aging, and disease. This section explores telomere replication and maintenance, the consequences of telomere shortening, and the implications of telomere dysfunction.
Telomere Replication and Maintenance
Telomere replication presents a unique challenge due to the inherent limitations of DNA polymerase, the enzyme responsible for copying DNA sequences. The end-replication problem arises because DNA polymerase cannot fully replicate the ends of linear chromosomes, resulting in the progressive loss of telomeric repeats with each round of cell division.
To counteract this problem, telomeres utilize a specialized mechanism called semi-conservative replication. Telomerase synthesizes and adds telomeric DNA repeats to the chromosome ends using its integral RNA template. This replenishes the lost telomeric sequence during replication, maintaining telomere length and chromosome stability.
In addition to telomerase, alternative mechanisms, such as recombination-based mechanisms known as alternative lengthening of telomeres (ALT), can elongate telomeres in the absence of telomerase. However, the precise details of ALT and its regulation remain the subject of ongoing research.
Telomere Shortening: Cellular Senescence and Aging
Despite the presence of telomerase, telomeres gradually shorten over time because its activity is limited or absent in most somatic cells. Telomere shortening acts as a molecular clock that marks the progression of cellular aging. When telomeres become critically short, they trigger a DNA damage response, leading to cellular senescence—a state of irreversible cell cycle arrest.
Cellular senescence serves as a protective mechanism against the proliferation of cells with damaged DNA or impaired function. However, the accumulation of senescent cells, a hallmark of aging, contributes to tissue dysfunction and age-related diseases. Telomere shortening and subsequent cellular senescence also play a role in the decline of regenerative capacity and tissue homeostasis.
Implications of Telomere Dysfunction
Telomere dysfunction can arise due to various factors such as excessive telomere shortening, defects in telomere-binding proteins, or alterations in telomerase regulation. Dysfunctional telomeres can result in genomic instability, chromosomal fusions, aberrant recombination, and activation of DNA damage response pathways.
Telomere dysfunction has been associated with several age-related diseases and conditions. Short telomeres and impaired telomere function have been linked to increased susceptibility to cancer, cardiovascular diseases, neurodegenerative disorders, and immune system dysfunction. Dysfunction can also contribute to premature aging syndromes known as telomeropathies, characterized by accelerated telomere shortening and the early onset of age-related symptoms.
Exploring the consequences of telomere dysfunction is crucial for understanding the underlying mechanisms of aging and disease. Unraveling the intricate interplay between telomere biology, DNA damage response pathways, and cellular senescence holds promise for the development of potential therapeutic approaches targeting age-related conditions.
Telomeres undergo replication and maintenance processes to replenish lost telomeric repeats. Telomere shortening over time leads to cellular senescence, contributing to tissue aging and age-related diseases. Dysfunction of telomeres can result in genomic instability and disease susceptibility. Studying telomere mechanisms and dysfunction provides valuable insights into cellular aging, disease progression, and potential avenues for intervention.
Historical Milestones in Telomere Research
Over the years, several key discoveries and accomplishments have contributed to our understanding of telomeres and their significance in cellular biology. This section highlights three important historical milestones that have shaped the field of telomere research, starting from the initial discovery of telomeres to the prestigious Nobel Prize recognition.
The Discovery of Telomeres: Hermann Muller's Groundbreaking Findings
The groundwork for understanding telomeres was laid by Hermann J. Muller, an American geneticist, in the early 1930s. Muller conducted experiments using the fruit fly Drosophila melanogaster and observed a phenomenon called "position effect variegation," whereby the expression of certain genes was altered depending on their proximity to chromosome ends. Muller hypothesized that there must be special structures at the chromosomal ends that regulate gene expression.
This hypothesis laid the foundation for further investigations into these chromosomal ends, eventually leading to the discovery of telomeres. Muller's groundbreaking findings provided crucial evidence for the unique nature and significance of these structures in chromosome integrity.
Recognition of Telomerase: A Paradigm Shift
In the 1970s and 1980s, researchers continued to unravel the mysteries of telomeres and made significant progress in elucidating the mechanisms involved in their replication and maintenance. However, it wasn't until the late 1980s that an important breakthrough occurred—a paradigm shift in telomere research.
Two key contributors to this milestone were Elizabeth Blackburn and Carol W. Greider. In 1985, Blackburn and Greider discovered an enzyme called telomerase in the single-celled organism Tetrahymena while studying telomeres and their replication. Telomerase was found to be responsible for adding telomeric DNA sequences to the ends of chromosomes, counteracting the progressive telomere shortening during DNA replication.
The discovery of telomerase opened up new avenues for understanding telomere biology, the regulation of telomere length, and its implications in aging and disease. It represented a breakthrough in our understanding of cellular aging processes, as well as potential therapeutic interventions.
Nobel Prize Award: Shaping the Field of Telomere Research
The groundbreaking discoveries made by Blackburn, Greider, and another key contributor, Jack W. Szostak, in the field of telomere research were widely recognized and awarded the Nobel Prize in Physiology or Medicine in 2009.
Their collective work revolutionized the understanding of telomeres, telomerase, and their significance in maintaining genome stability and cellular health. The Nobel Prize laureates' research shed light on the critical role telomeres play in cellular aging, disease development, and potential interventions. This esteemed recognition further elevated the field of telomere research, fostering increased interest, advancements, and collaborations in the pursuit of knowledge and practical implications.
The Nobel Prize award significantly influenced the field of telomere research, attracting more scientists to explore this intriguing realm of cellular biology. The impact of these remarkable discoveries continues to shape our understanding of aging, disease, and the potential for targeted interventions to promote healthy aging.
Telomeres and Aging
Telomeres play a vital role in the aging process, and comprehending their significance provides insights into various age-related phenomena and diseases. This section delves into the mechanisms by which telomeres serve as a measure of cellular aging, explains the concept of the Hayflick limit and cellular clocks, and explores the connections between telomeres and age-related diseases.
Telomeres as a Measure of Cellular Aging
Telomeres serve as a measurable indicator of cellular aging due to their progressive shortening with each round of cell division. Several factors influence telomere length, including genetic predisposition, lifestyle choices, and environmental factors. As telomeres gradually erode over time, cells reach a critically short telomere length, leading to cellular senescence or cell death.
Telomere length has gained significant attention as a potential biomarker for cellular aging. It reflects the combined effects of intrinsic and extrinsic factors on an individual's cells, providing insights into the biological age of tissues and organs. Accelerated telomere shortening has been associated with an increased risk of age-related diseases and overall mortality.
Studies have showcased correlations between telomere length and age-related disease risks. Individuals with shorter telomeres generally exhibit a higher likelihood of developing age-related diseases and experiencing adverse health outcomes. Thus, telomeres serve as potential indicators for disease susceptibility and may assist in assessing individual health and disease risks.
Telomeres: The Hayflick Limit and Cellular Clocks
The Hayflick limit, named after Leonard Hayflick, refers to the maximum number of times a cell can divide before reaching replicative senescence. This limit is believed to be influenced by telomeres and their progressive shortening with each cell division. Once telomeres become critically short, cell division ceases, and the cell enters a state of permanent growth arrest, referred to as cellular senescence.
Telomere shortening serves as a type of cellular clock that impacts the lifespan of cells. It regulates the proliferative capacity of cells and helps prevent the accumulation of errors and damaged DNA. As telomeres erode, the cell's ability to divide diminishes, ultimately contributing to tissue aging and the finite lifespan of organisms.
The concept of cellular clocks underscores the importance of telomeres in the aging process. Telomere length provides a biological timer that helps dictate cellular and tissue lifespan, impacting overall organismal aging and health.
Linking Telomeres to Age-Related Diseases
Telomere dysfunction has been implicated in various age-related diseases. The shortening of telomeres and impairment of telomere function contribute to genomic instability, cellular senescence, and tissue dysfunction. This link has been observed in diseases such as cardiovascular disorders, neurodegenerative conditions (e.g., Alzheimer's disease), certain cancers, and immune system dysregulation.
Studies have provided evidence for associations between telomere length and age-related disease risks. Individuals with shorter telomeres tend to have an increased likelihood of developing age-related diseases and experiencing poor health outcomes. Telomeres, therefore, serve as potential prognostic indicators for disease susceptibility and have the potential to enhance risk assessment and disease prevention strategies.
Understanding the implications of telomere dysfunction in age-related diseases offers valuable insights into disease mechanisms. It highlights the significance of telomeres as potential therapeutic targets and allows researchers to explore interventions aimed at restoring or preserving telomere integrity to mitigate the effects of aging-related conditions.
Telomeres and Anti-Aging Strategies
Understanding the impact of telomeres on aging has fueled the exploration of various anti-aging strategies focusing on telomere maintenance and preservation. This section discusses the potential of activating telomerase as an anti-aging approach, the challenges associated with telomere-lengthening therapies, and emerging research directions for telomere-related anti-aging interventions.
Activating Telomerase: Potential Anti-Aging Approach
As telomerase can counteract telomere shortening, activating this enzyme has emerged as a potential anti-aging strategy. By enhancing telomerase activity, it may be possible to prevent or reverse telomere shortening, providing therapeutic benefits for age-related conditions. However, regulating telomerase activity remains a complex challenge, as uncontrolled telomerase activation can lead to uncontrolled cellular proliferation and tumorigenesis.
Various approaches are being explored to selectively activate telomerase while maintaining strict control over its activity. These strategies aim to strike a balance between addressing telomere shortening and avoiding potential risks associated with unrestricted cell growth. Developing precise molecular tools or therapeutic interventions to activate telomerase in a controlled manner remains an area of ongoing research.
Telomere Lengthening Therapies: Challenges and Potential
Researchers are investigating various approaches to develop therapies that lengthen telomeres. Small molecule interventions, gene therapies, and stem cell-based regenerative medicine are being explored for their potential to counteract telomere shortening and promote healthy cellular aging. However, significant challenges exist, including the need for targeted delivery mechanisms, balancing efficacy with potential risks, and ensuring the long-term safety and sustainability of any interventions.
Achieving telomere lengthening requires extending telomeric DNA specifically without triggering detrimental effects such as genomic instability or tumorigenesis. Developing telomere-lengthening therapies that effectively and safely rejuvenate aging cells and tissues remains a complex task and an active area of investigation.
Emerging Research and Future Directions
Research on telomeres and their role in aging continues to advance, exploring the intricate mechanisms of telomere biology and its potential implications for age-related processes and diseases. Emerging areas of research include the modulation of telomerase activity, the development of telomere-targeted therapeutics, and the exploration of lifestyle factors that influence telomere health.
Future directions in telomere research hold promise for advancing our understanding of aging processes and potentially revolutionizing the field of anti-aging interventions. With further insights into telomere dynamics, researchers may identify novel strategies to enhance cellular health, delay aging, and mitigate the burden of age-related diseases.

Here are some research papers.
Blackburn, E. H., & Gall, J. G. (1978). A tandemly repeated sequence at the termini of the extrachromosomal ribosomal RNA genes in Tetrahymena. Journal of Molecular Biology, 120(1), 33-53.
Cech, T. R. (2004). Nobel Lecture: Self-splicing RNA: implications for evolution. Bulletin of the American Mathematical Society, 41(3), 345-360.
de Lange, T. (2005). Shelterin: the protein complex that shapes and safeguards human telomeres. Genes & Development, 19(18), 2100-2110.
de Lange, T. (2018). Shelterin-Mediated Telomere Protection. Annual Review of Genetics, 52, 223-247.
Greider, C. W., & Blackburn, E. H. (1985). Identification of a specific telomere terminal transferase activity in Tetrahymena extracts. Cell, 43(2), 405-413.
Blackburn, E. H., & Greider, C. W. (2006). Telomeres and telomerase: the path from maize, Tetrahymena and yeast to human cancer and aging. Nature Medicine, 12(10), 1133-1138.
de Lange, T. (2009). How telomeres solve the end-protection problem. Science, 326(5955), 948-952.
O'Sullivan, R. J., & Karlseder, J. (2010). Telomeres: protecting chromosomes against genome instability. Nature Reviews Molecular Cell Biology, 11(3), 171-181.
Blasco, M. A. (2017). Telomeres and human disease: aging, cancer, and beyond. The Journal of Clinical Investigation, 127(3), 996-1002.
Harley, C. B. (2011). Telomerase, aging, and cancer: In search of a happy ending. Oncogene, 30(2), 137-140.
Sanders, J. L., Newman, A. B. (2013). Telomere length in epidemiology: a biomarker of aging, age-related disease, both, or neither? Epidemiologic Reviews, 35(1), 112-131.
Sahin, E., & Depinho, R. A. (2010). Linking functional decline of telomeres, mitochondria and stem cells during ageing. Nature, 464(7288), 520-528.
Shay, J. W., & Wright, W. E. (2019). Telomeres and telomerase: Three decades of progress. Nature Reviews Genetics, 20(5), 299-309.
Armanios, M., & Blackburn, E. H. (2012). The telomere syndromes. Nature Reviews Genetics, 13(10), 693-704.
Muller, H. J. (1938). The remaking of chromosomes. Collecting Net, 13(3), 181-198.
Blackburn, E. H., & Greider, C. W. (1985). Identification of a specific telomere terminal transferase activity in Tetrahymena extracts. Cell, 43(2), 405-413.
de Lange, T., Lundblad, V., & Blackburn, E. H. (2006). Telomeres. Cold Spring Harbor Perspectives in Biology, 4(12), a005579.
Nobel Prize. (2009). Nobel Prize in Physiology or Medicine 2009. Retrieved from https://www.nobelprize.org/prizes/medicine/2009/summary/
Bengtsson, L. (2010). The discovery of telomeres and telomerase: an outline of our current understanding of human telomeres and telomeropathies. Dissertations in Molecular Medicine, 1(5), 1-38.